The aim of this project is to create a remote control system for a robotic arm controlled by using the Kinect v2 sensor, to track the movements of the user arm, without any additional point of measurement (marker-less modality).
The Kinect camera acquires a 3D point cloud of the body and a skeleton representation of the gesture/pose is obtained using the SDK library software provided by the Kinect. The skeleton joints are tracked and used to estimate the angles.
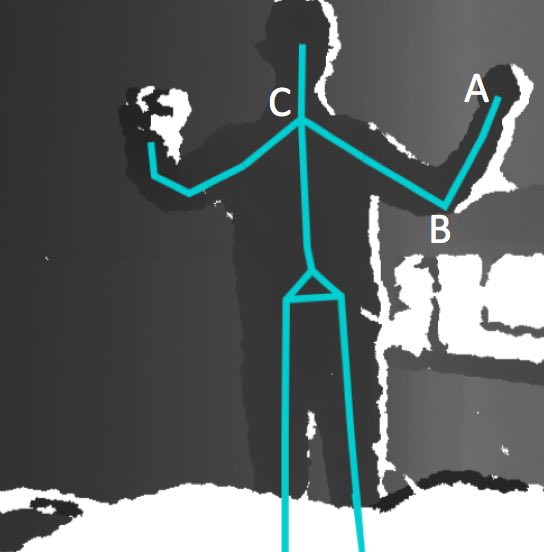
Point A is the joint of the wrist, point B is the joint of the elbow and point C is the joint of the shoulder. In the three dimensional space, vectors BA and BC are calculated with using the space coordinates of points A, B and C, which are taken from the skeleton. Angle α is calculated by using the dot product of the two vectors.
The software has been developed in C# in Visual Studio 2015.
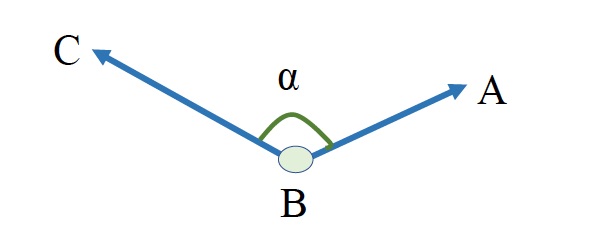
Related Publications
Sarikaya, Y.; Bodini, I.; Pasinetti, S.; Lancini, M.; Docchio, F.; Sansoni, G. “Remote control system for 3D printed robotic arm based on Kinect camera“, Congresso Nazionale delle Misure Elettriche ed Elettroniche GMEE-GMMT. 2017